Movement
The universe, and everything in it, is continuously moving and changing. Objects fall, planets rotate, plants and animals move and atomic particles are in a continuous state of movement and change.
Movement is the change in position of an object over time, from one place to another. Movements can be fast or slow. Students are curious about how they move themselves and how they can make other things move.
Movement is a concept that assists students to make sense of the world around them. In the early years, students are developing gross and fine motor skills. They are interested in what they can do and how they can do it. They have growing skeletons and muscles that allow them to move in many different ways, such as jumping, sliding, rolling and spinning.
Forces
Forces change the way things move. Forces can make stationary objects move and moving objects speed up, slow down or stop. Forces can change the direction an object is moving and can even change its shape. It is not possible to ‘see’ a force—it is only possible to feel or observe its effects.
Examples of forces include pushes, pulls, friction, gravity and magnetism. A force can be applied to an object but is not a property of the object itself.
Put simply (and as a handy rhyme):
Forces move things in their way:
They can also make them stay
Speed them up or make them slow
Forces change the way things go.
Forces also make things change:
Make them bend or break or twist,
Make their shapes look really strange
Stretch them out or squash them—squish!
Forces can act through direct contact, such as physical pushes and pulls, friction, and air or water resistance. The force exerted by a magnet acts at a distance. This means that a magnet does not have to touch an object to exert a force on it. There are other forces that act at a distance, including gravity and static electric force. Magnets cannot exert a force on an object that is too far away.
Force has two aspects: magnitude and direction. The magnitude of the force refers to the size or amount of force exerted, for example, a strong or a weak kick (push force) to a soccer ball.
Force-arrow diagrams
Force-arrow diagrams are used to represent the direction and the size of forces acting in a particular situation. The length of a force-arrow represents magnitude and the direction of the arrow shows the direction in which the force is acting. When drawing force-arrow diagrams, longer arrows in the direction of the force are used to represent a larger force, while a shorter arrow in the direction of the force is used to represent a smaller force. Asking students to label the force-arrows will assist with their representations and explanations of forces and motion.
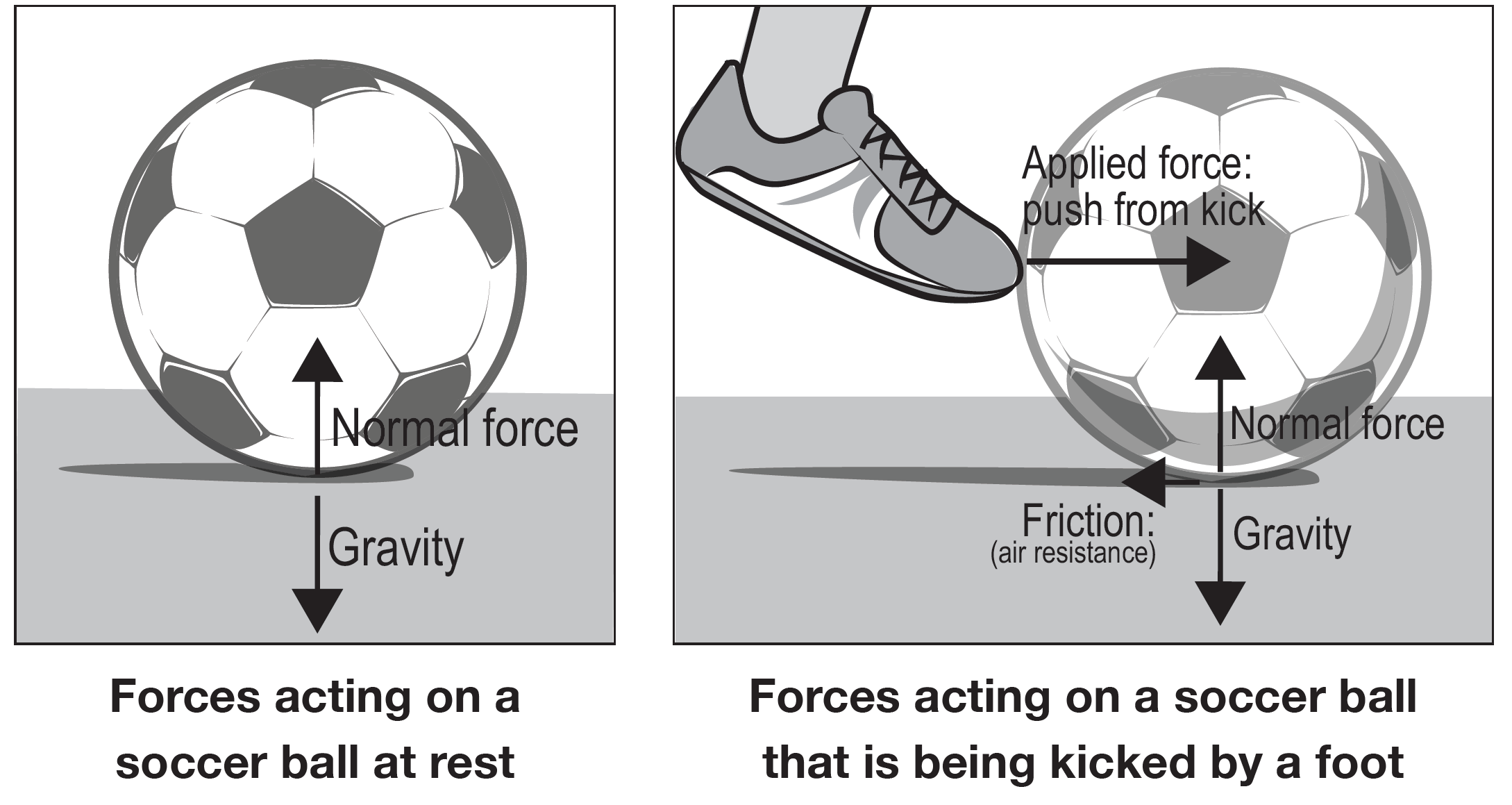
Note: The diagrams above are not force-arrow diagrams in the strictest sense, as scientists would portray all forces acting on an object’s centre of mass. It is also not what you would expect students to be able to draw. The concept of normal force (which refers to a force perpendicular to the two surfaces in contact, generally as a result of resisting gravity) is formally introduced at secondary school.
Opposite forces
Forces come in pairs—one force is an action while the second force is a reaction. For example, our standing body is pulled down to the ground and the ground pushes back against our feet. We are able to be stationary because even though there are two forces acting on us, these forces are of equal magnitude in opposite directions. The forces are therefore said to be balanced.
The balance between opposite forces determines whether objects float or sink in water, and whether objects in air fall quickly like a stone, slowly like a feather, or float like a hot air balloon.
Marbles roll when pushed and a toy car rolls down a slope if the pull of gravity is greater than the force of friction that opposes its movement. As the car can’t move straight down towards the pull of gravity, because of the friction it accelerates down the slope more slowly than if it were dropped from the same height. The steeper the slope, the faster the car will roll down it.
Paper planes will glide through air with the air pushing them up, opposing the pull of gravity, until they are slowly pulled down to the ground. Toy boats and balls will float on water that pushes on them from below, opposing the pull of gravity.
A stationary object has forces acting on it, but it is stationary because those forces are balanced. For example, a soccer ball at rest (see force-arrow diagram above left), remain stationary because there are two forces of equal magnitude acting on them (the pull from gravity and the opposing push from surfaces (normal force)). To start moving to a desired speed, a stationary object requires an imbalanced force to be applied, for example, the stationary soccer ball receives a kick (see diagram above right). Once that speed is reached, it only requires a small push to balance out forces that are slowing it down, such as air resistance and friction.
Mass and magnitude
Forces of different magnitude have different effects on different objects. For example, a big push will make a swing move a lot while a small push will only make the swing move a little. The effect of a force on an object also depends on the object’s mass, which is the amount of matter in the object. For a given force, an object with a smaller mass will experience a greater effect than one with a larger mass. For example, a small push on a light wooden block will make it move a long distance, while the same small push on a heavy wooden block will not make it.
Contact forces
Contact forces result from two objects being in physical contact with each other. Friction is an example of a contact force. Other contact forces include normal force (the upward force of a desk on a book resting upon it) and applied force (the force exerted on a shopping trolley as it is pushed).
Friction
When an object is moving over a surface, the contact between it and the surface creates friction. The friction force opposes the direction of the movement of the object, slowing it down.
Friction occurs between all types of materials whether they are solids, liquids or gases. Friction is caused by the interaction between surfaces causing them to ‘stick’ together and oppose the movement between them.
To make something move, we need to apply enough force (transfer enough energy to the object) to overcome the force of friction opposing that movement. Moving objects slow down and stop because the force of friction acts against the movement (changes the movement energy to heat, and sometimes sound energy) and slows the object to a stop.
A friction force opposes the direction of movement, so when depicting friction on force-arrow diagrams the friction force-arrow is drawn in the direction opposing the motion and will always be smaller or equal to the push/pull force.
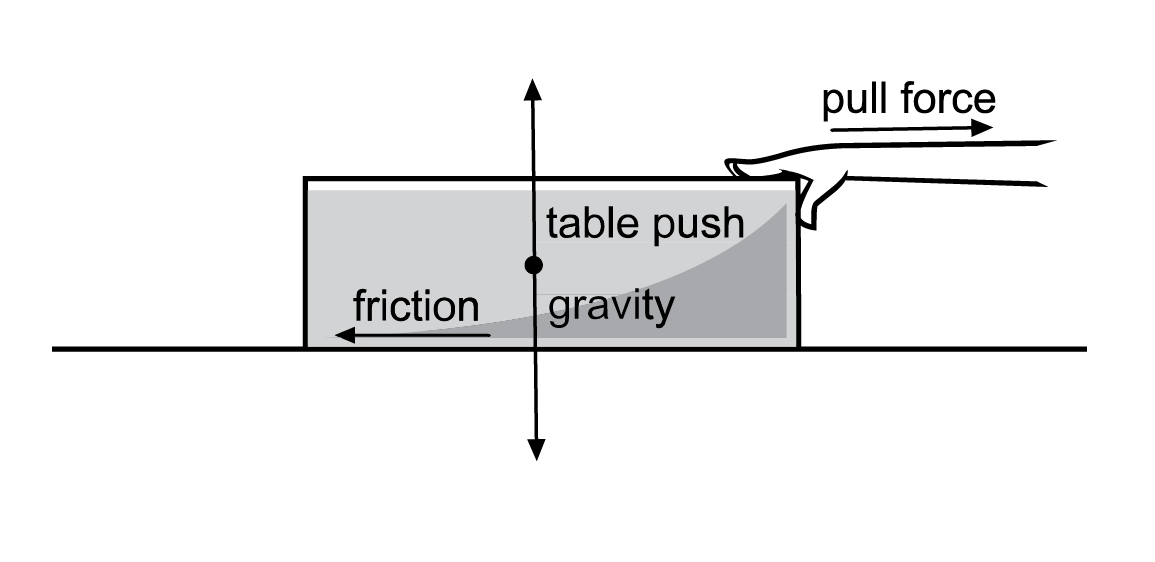
Diagram of forces including friction
Scientists and engineers try to find ways to both increase and decrease friction. This is because there are situations where friction is helpful and other situations where friction is a problem for us. Think about trying to walk on wet kitchen tiles in bare feet (more friction would help) and then trying to slide down a slippery dip with wet trousers (less friction would help).
Everyday applications of friction include:
- allowing us to move: the soles of our shoes grip the ground and help us to push off when walking
- slowing things down: the brakes on a bicycle decrease the speed of the bicycle and heat the wheel, while friction between the tyre and the road stops the bicycle from simply sliding along
- keeping things in place: tuning pegs on guitars and violins stay in place and keep the instrument in tune, nails hold materials together and objects on a gentle slope do not slide
- transforming movement enery into heat energy: it can be used to create fire, for example, by rubbing sticks together.
Friction can have some disadvantages. Frictional forces cause surfaces to wear: the soles of shoes wear down, tread on tyres wears down, moving parts in machinery wear down and need to be replaced. Friction generates heat and this can be a problem if things get too hot.
Ways to reduce friction include:
- adding a lubricant: a liquid such as water, oil or detergent makes surfaces slippery
- reducing the force pushing the two objects together: a lighter object is easier to move across a surface than a heavy object.
Momentum
If an object is moving, it is also said to have momentum. The more momentum an object has the harder it is to stop. Just as an object requires a large force to make it travel quickly, a fast-moving object will require a large force to stop it moving. For example, when we run, we have more momentum than when we walk, hence a bigger force is needed to make us stop. The same is true for cars; it is easier to stop a car when it is moving slowly than when it is moving fast. This is why slower speed limits are in place around areas where there might be lots of people, such as schools, hospitals and shopping centres.
Objects with more mass require a larger initial force to move at the same speed as objects with less mass. Similarly, a more massive object will require a larger force to stop it moving than an object with less mass travelling at the same speed. For example, at a given speed, stopping a bicycle requires less force than stopping a car, and both require less force than stopping a truck.
Friction between the object and the surface it moves over also makes a difference to how hard or easy it is for an object to stop. For example, it is easier to stop running on a tarred road than to stop sliding on ice.
Gravity
Isaac Newton is claimed to have said, ‘What goes up, must come down’ when he saw an apple fall from the tree he was sitting beneath. He is one of the scientists who have developed our understanding of forces, including gravity.
Every object exerts a gravitational force on other objects but it can be hard to detect unless at least one of the objects has a large mass. Mass is a measure of the amount of matter an object has while weight is the measure of gravitational pull that acts on an object. The weight of an object is related to the mass of the object and the magnitude of the gravitational force acting on the object.
The weight of an object will change if the gravitational force acting on it changes, but its mass will not. For example, the Moon is not as massive as the Earth so its gravitational force is not as strong. Because of this, objects will not be attracted as strongly to the centre of the Moon as they would be to the centre of the Earth, and their weight on the Moon is less than their weight on Earth.
The Earth has such a large mass that the gravitational attraction between it and most things is very noticeable; when we jump into the air, the Earth’s gravitational force pulls us back towards the Earth’s centre very quickly. We can also feel the pull of the Earth’s gravity when we try to lift things; the more mass something has, the greater the pull of gravity and the greater the lifting force we need to use.
Gravity acts on an object regardless of whether or not the object is moving. It does not require the object to be surrounded by air or water or anything else and can therefore act in the vacuum of space.
Magnets
A magnet is a material that generates a magnetic field. Magnets only attract materials composed of iron, nickel and cobalt. These materials are described as being magnetic. Steel is attracted to magnets as it is mostly made of iron. Most other materials such as wood, plastic, aluminium, silver and copper are not attracted to magnets and are called non-magnetic.
Large magnets are generally stronger than smaller ones made of the same material and shaped in the same way. However, magnets made of different materials have differing degrees of magnetic force. Rare-earth magnets are strong permanent magnets made from alloys of rare earth elements. They produce significantly stronger magnetic fields than other types of magnets so a smaller rare-earth magnet will have stronger pull than a larger ‘common’ magnet.
When the N poles of two magnets are brought together, the magnets will be repelled—that is, they will move away from each other. The same thing happens when the S poles are brought together. When the N pole of one magnet is brought near the S pole of another, the two magnets will strongly attract each other and will move toward each other. This can be summarised as ‘like poles repel, unlike poles attract’.
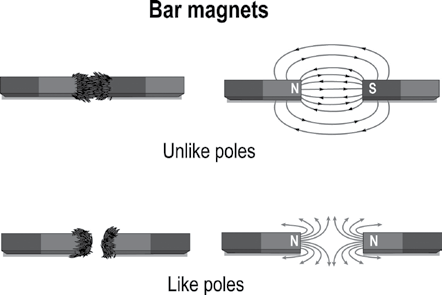
Like poles repel, unlike poles attract
Magnets play an important yet often unseen role in many everyday objects:
- Vacuum cleaners use powerful magnets in their motors to give high suction.
- Telephones use small magnets in their microphones and speakers to capture and emit sound.
- Smart phones use small magnets to interact with a coil of wire to create the vibrate function.
- Kitchen cupboards have magnetic catches to keep the doors closed.
- Microwaves usually have two magnets in their Magnetron to guide the electrons to heat the food.
- Electric can openers use a magnet to hold onto the lid as the can is opened.
- Recycling plants use huge magnets to sort scrap metal.
A magnetic field is the region around a magnet in which the magnet exerts force. Many small pieces of iron, called iron filings, are used to show the magnetic field around a magnet. The iron filings form a pattern of lines called magnetic field lines. The arrangement of the magnetic field lines depends on the shape of the magnet, but the lines always extend from one pole to the other pole. The force is weaker farther away from the magnet.
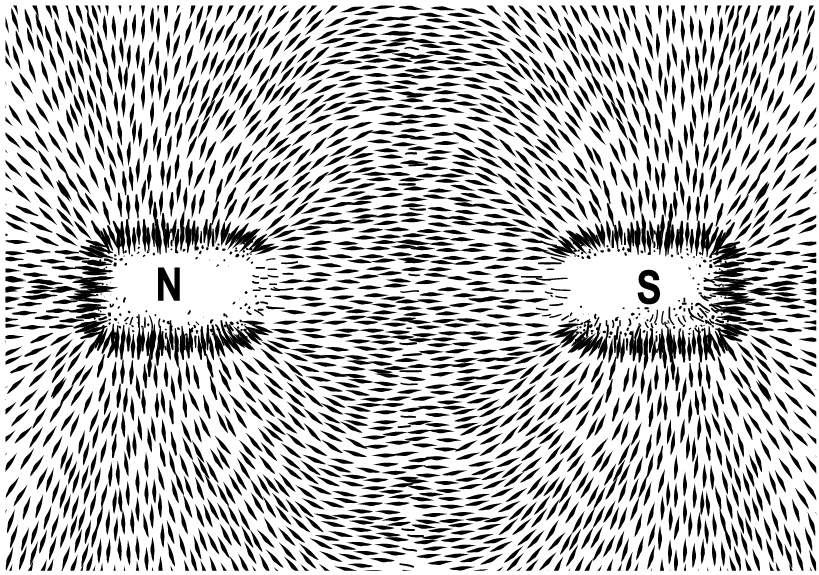
Iron filings can be used to show the magnetic field surrounding magnets
Linking force and energy
When forces are applied to an object, energy is transferred or transformed. When a football player kicks a ball, movement energy in their foot is transferred to the ball. When hands are rubbed together, friction transforms movement energy into heat energy. When you turn on a switch, electrical energy from the power station is transformed into light energy in your room.